The Detection Problem: An Eight-Decade Challenge.
The Difficulty of Practically Detecting and Discriminating Mines, Booby Traps, and Victim Operated Improvised Explosive Devices
CISR JournalThis article is brought to you by the Center for International Stabilization and Recovery (CISR) from issue 28.1 of The Journal of Conventional Weapons Destruction available on the JMU Scholarly Commons and Issuu.com.

By Roly Evans, Tracey Temple, PhD, and Lt Col (Retd) Liz Nelson [ Cranfield University ]
Reliably detecting and discriminating mines, booby traps, and victim operated improvised explosive devices (IEDs) remains a stubborn problem for both humanitarian demining organizations and the military. Since mines were widely used during the Second World War, much effort has been expended on the detection problem, with limited success. The aim of being able to positively identify a device first time remains elusive since the scientific challenge of positively identifying different substances in the ground is formidable. This article critically examines the detection problem and suggests that in the continued absence of a ‘silver bullet’ technological solution, the best means currently available to manage the risk of concealed explosive devices is the systematic collection and analysis of relevant operational data from the field.
Introduction
Neither military nor humanitarian actors can reliably detect and discriminate mines and booby traps in operational environments. This has been the case since mines became a common feature of conflict during the Second World War.1 There have been many claims that a given sensor is the answer and can not only detect an item but discriminate it from everything else that is likely to be found, whether it is fragmentation from exploded ordnance (EO), other metal contamination, various soil types, or even rocks.2,3,4,5,6,7,8 However, despite the assertions made over eight decades, military units and humanitarian demining organizations still struggle to reliably detect and discriminate victim operated explosive devices (VOEDs), including IEDs,9 anti-personnel (AP) mines,10 anti-vehicle (AV) mines,11 and booby traps.12 Mines or IEDs with minimum metal content provide an extra level of detection and discrimination difficulty. Even explosive remnants of war (ERW)13 with significant metal casings are hard to discriminate from false positives in metal contaminated ground and therefore effectively detect. Difficulty in detection and discrimination makes search and clearance painfully slow in both humanitarian and military contexts.
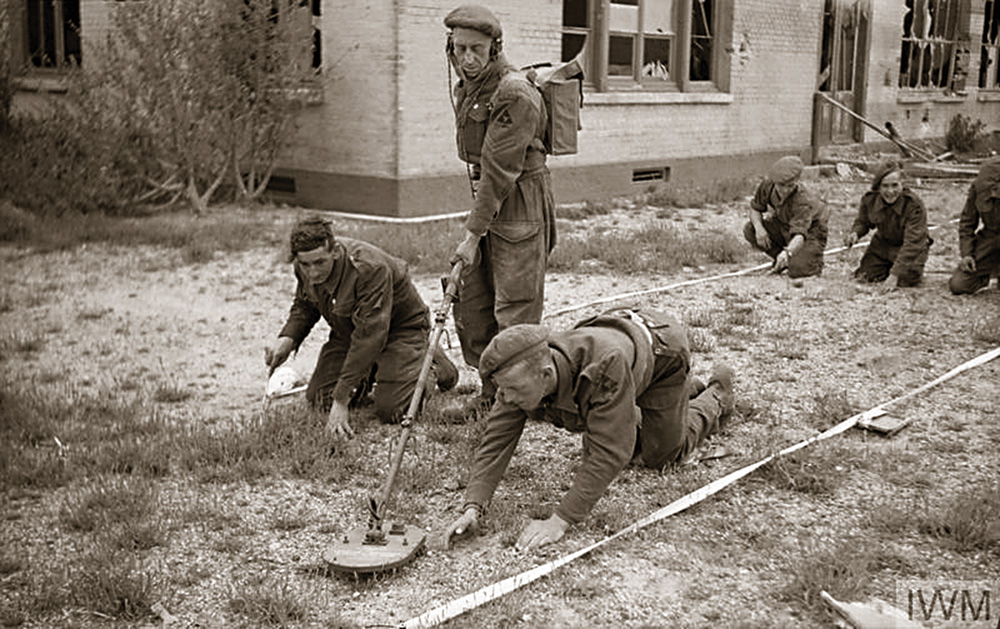
This article will critically analyze what has sometimes been referred to as the “detection problem.”14,15,16 The lack of a technological solution is naturally a key explanation as to why it has not been solved, but the reasons are more complex. Sometimes the problem has not been properly defined into a well-formed requirement that acknowledges the need for a reliable detection capability in operational conditions. Overly positive claims of solutions have been commonplace. Added to this has been an unwillingness, at least in military circles, to remain fully engaged with the problem except during times of conflict, and especially during counter insurgencies when its urgency means it can no longer be ignored.17 The technology might have fallen short in meeting the mine detection problem, but in some ways so has the military18,19,20 and humanitarian21,22,23,24 approach.
This is important because the detection problem matters. It matters since the progress of humanitarian efforts to clear land and enable development in post-conflict environments is significantly impeded by the difficulty of practically detecting and discriminating VOEDs.25,26 It matters since IEDs, many of them victim operated, are a key, possibly the key means of inflicting casualties during an insurgency, to the extent that their use was in 2006 deemed to have strategic significance.27 It matters since mines and booby traps are still a significant aspect of modern conventional warfare.28,29,30,31
The Detection Problem
On 18 February 1946, a meeting took place in the Ministry of Supply in the Shell Building on the Strand in London, attended by senior military officers, civil servants, and research scientists. The purpose of the meeting was “research into the clearance and detection of landmines.” After years of various means of detection development, from early Soviet and Japanese efforts in the late 1930s,32 and French efforts in 1940,33 followed by extensive US34 and British35 research before and throughout the war, General Sir Eustice Tickell, the British Army Engineer in Chief stated “that at present the Army had no answer to the mine problem.”36 A 1946 UK Signals Research and Development Establishment (SRDE) memorandum noted that “the mine was at times during the war the limiting factor in retarding mobility and came to be regarded as the Queen of the Battlefield.”37 Another Ministry of Supply letter noted that, “you will no doubt fully appreciate the vital nature of this problem so far as the Army is concerned.”38 In an infantry conference in Fort Benning in the United States in June 1946, it was recognized that despite claims of being able to detect at least mines with metallic content, “the means available during the past war for mine detection consisted principally of manual probing and hand carried mine detectors.” The latter “had many deficiencies.”39
Six decades later in early 2006 the Joint Improvised Threat Defeat Organization (JIEDDO) was established with a recognition that to defeat IEDs, soldiers must first be able to detect them.40 In 2005, Lt General John Abizaid sent a memo to the Under-Secretary of Defence calling for a Manhattan Project-like effort to counter IEDs.41 In 2008, it was admitted that “in the absence of a reliable method to detect IEDs and prevent their use, Coalition Forces have dedicated significant efforts and resources to searching, clearing and securing roads and other vital areas.”42 In 2011, “after five years of work, hundreds of projects, and a blizzard of cash paid to some of America’s biggest defence contractors, JIEDDO has not found a high-tech way to detect” IEDs. “In fact, the rate at which soldiers are able to find IEDs before they explode has remained mostly steady, at roughly 50 percent, since JIEDDO was formed.”43 The then outgoing JIEDDO director, Lt. General Michael Oates, stated that “the best bomb detectors are still dogs working with handlers, local informants, and the trained soldier’s eye.”44 The following year, after over a decade of counter insurgency in Afghanistan, it was observed that “despite spending billions of dollars on detection technologies, the United States has yet to develop an effective long range stand-off detection capability and the find rate prior to detonation has remained at around 50 percent. The best methods of detection remain mine detection dogs, humans and low-tech tools.”45
The last eight decades have seen significant progress in military technology. Some believe this represents a revolution in military affairs;46 however, one technological problem—the detection problem—has proved practically unsolvable. Tools that are available, such as metal detectors, have become more refined, to the point of being declared a “mature technology.”47 However, the fundamental problem of not only detecting VOEDs, but also discriminating them from many other false positives remains.48,49 Technology that might look promising in a laboratory or in favorable test conditions has consistently fallen short in an operational environment. The aim of positively identifying a VOED first time remains elusive.
Detecting What?
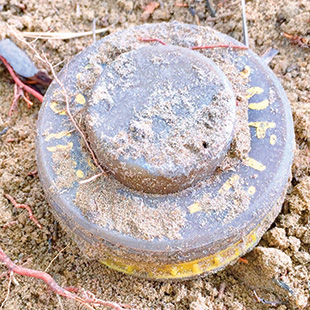
What is available to be detected in a mine, booby trap, or victim operated IED? For the first mines, and even for many mines deployed today, the metal content was the obvious material, whether it was part of the casing or the fuzing system. As minimum metal mines developed during the Second World War, wooden, concrete, clay, tar, or even glass casings became common. Now the metal components can be as little as an aluminum cup for the detonator. In the 1980s, the US National Ground Intelligence Center defined a minimum metal mine as having 2.46g of metal.50 This was the amount of metal in the M606 fuze assembly of the M-19 minimum metal mine. The only metal was a minimal part of the striker and the aluminum detonator capsule.51 In some minimum metal mines, such as the Spanish P-4-B, even the striker is made of plastic, with the only metal being a chromium spring that acts as a holding device, and the detonator capsule with a foil cover for the primer.52 Perhaps the only component of a blast mine that is still invariably metal is the detonator capsule or primer cover (aluminum),53 with it being viable to manufacture other components from non-metallic materials. Devices with an electrical means of initiation, such as many victim operated IEDs, will typically have a battery as a power source and this, depending on the depth it is buried, is as detectable as other significant metal components. Other objects associated with electrical devices, such as an aluminum detonator casing, are usually more detectable than the thin copper wiring used to connect the circuit. Mechanically initiated victim operated IEDs and booby traps can in theory have no metal content but in practice consistently do.54
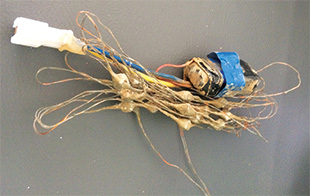
Many items of EO, whether found as ERW55 or employed as main charge components in an IED or booby trap, will have substantial metal content. A significant proportion of EO employs a metal casing not only to provide fragmentation but also to withstand the extreme forces applied on firing or dropping. If used as a main charge in a victim operated IED, it can make that device more detectable, although it is not always this simple. Such devices might be at a distance from the switch that initiates the device, which itself might not be easily found. There are mines that rely on primary fragmentation as their lethal mechanism, such as directional, omnidirectional, and bounding fragmentation mines. These, and booby traps that employ an explosive fragmentation munition such as a grenade, naturally have metal casings that are in theory more detectable but sometimes in practice can still present a challenge.56,57 In all cases, in areas of metal contamination, all EO is very difficult to practically detect since it is hard to discriminate from other metal clutter. This will result in searchers investigating a high proportion of false positive signals.
Alternatively, a detector can try to detect the explosive charge itself, especially for devices the size of an AV mine or equivalent IED, the charge represents sufficient material that can have an identifiable density different than the surrounding soil. Chemical detection of the explosive is also theoretically possible, especially if the case is ruptured. Mines may also be differentiated from the surrounding soil by a differing heat signature. The fundamentals of what components are common to all concealed explosive devices were identified during efforts to address these weapons during and after the Second World War. During the Ministry of Supply meeting in February 1946 it was “recognised that an explosive and detonator were the only inescapable components of any mine, and that any casing (metallic, magnetic or plastic) and any trip mechanism (operated by pressure, displacement, magnetism, electrical induction, delay, remote control etc.) were variable incidentals.”58
Electro Magnetic Induction Detectors
The Soviets can possibly lay claim to the first widescale operational use of a magnetic mine detector during the Winter War of 1939–1940.59 For the United Kingdom, the first operational use of a ‘metal detector’ to detect mines was the deployment of the Goldak commercial metal detector in 1941, soon to be replaced by the Polish mine detector (PMD) in the western desert by October 1942. Minefields are obstacles and warfare in the open desert proved a catalyst for the development and deployment of mines as a means of creating artificial obstacles. Almost all mines in 1942 employed metal in both the casings and the fuzing system. The detector employed two coils connected to an oscillator, the current of which generated an oscillating acoustic frequency connected to headphones by means of an amplifier. The charge in each coil was balanced but this balance was altered when proximity to metal caused impedance. The detector went through three versions until the No.4A, which added a regenerative circuit. The No.4C became the standard for decades beyond end of the war.60 These detectors were also widely used in the clearance of metallic mines in the United Kingdom and Europe.61 Concurrent to this, the United States developed the Detector Set, Anti-tank Mine, Portable SCR-625 in early 1940.62 This would become the standard US detector of the conflict. Similar in concept to the PMD, but with three coils rather than two, the SCR-625 also operated on the principle of a “balanced mutual inductance bridge.”63
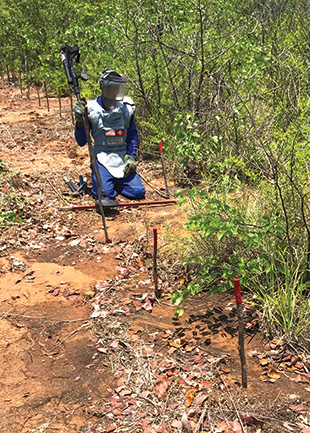
While electro magnetic induction (EMI) detectors became the standard means of searching for mines with sufficient metal content, they were not always the primary means, due to their limitations. In 1962, over twenty years after detectors were first deployed, their practical limitations were well known to the Royal Engineers. While conceding that ‘electronic detectors’ can search ground far more rapidly than prodding, the official Detection and Clearance of Mines Pamphlet noted that the metal that may be found in mines was “not clearly distinguishable in the head-phones of a detector from other small metallic objects likely to be present in the ground, particularly on the battlefield. The use of detectors is therefore confined to following up prodders to search for deep buried metallic mines which are too deep for the prodders and any shallow metallic mines which they may have missed.”64 The same approach was also adopted by the US military.65
In the decades since, EMI detectors have become increasingly refined and have remained the mainstay of efforts to detect explosive devices with at least some metal content, including some minimum metal landmines. Deemed a “mature technology” in 2006, they remained “the primary means of detection used in mine action programmes,”66 rather than a follow-up technique for prodding which even in 1962 was recognized as “not a safe method.”67 The fundamental design retains transmitter and receiver coils, with a time varying current creating a low frequency electromagnetic field that can induce eddy currents in a nearby metallic object. Modern metal detectors have been labelled “low frequency EM induction devices.”68 Some soils, such as laterite soils in Southeast Asia, can also induce current in the receiver coil. Much of the development in EMI detectors has been focused on the use of algorithms to discount such signals. Modern detectors might have a ground compensation feature that is activated in a clear piece of ground but enables the detector to register the electromagnetic signature of the soil in order to subsequently discount it. This is known as soil signature suppression.69 No answer to significant metal contamination has yet been found. Fragmentation from detonated EO means many false positives, all of which have to be investigated by the deminer. Sometimes the density of false positives in an area invalidates the use of a detector and the only remaining option is full excavation of the entire topsoil to a given depth (typically 13–20cm). EMI detectors also struggle near large concentrations of metal, such as vehicles, power lines, fences, and even reinforcement bars in walls and floors. While such detectors therefore remain a useful tool to assist in the search for VOEDs, the practical inability to discriminate signals means such sensors cannot be deemed a reliable solution to the detection problem.
Ground Penetrating Radar
Ground penetrating radar (GPR) makes use of the electro-magnetic spectrum, typically between 300 MHz70 and 2.6 GHz. The focused application of pulsed energy in this range, (more modern GPR may apply energy at around 1 GHz), is now common in GPR employed for demining purposes. Anomalies relative to the surrounding soil in the reflected signal can possibly indicate the presence of a mine but can also indicate the presence of a rock or a root. Electromagnetic waves can dissipate in saturated ground,71 and for this reason the effectiveness of GPR in such conditions is known to be very limited. As was recognized in February 1944 by a British scientist researching ultra-high frequency (UHF) detection, “the problem of locating buried non-metallic mines is one of great difficulty mainly because of the low contrast which exists between the mine and the surrounding earth in respect to most properties.”72
Modern applications of GPR tend to employ it in combination with an EMI detector as the primary means of detection. While this combination cannot categorically detect or discriminate concealed explosive devices, and is limited to the initial EMI indications, it can potentially reduce the false positive rate significantly. The US Department of Defense Humanitarian Demining Research and Development Program employed this concept with its Handheld Standoff Mine Detection System (HSTAMIDS). Published operational evaluation results from March 2006 to July 2021 in Cambodia, Sri Lanka, and Kosovo suggest that out of 33,437,336 detections made by the EMI sensor, 95.04 percent were rejected by the GPR sensor. Of the remaining 1,816,199 signals that were investigated, 56,907 (3.13 percent) were found to be mines.73 Applications of GPR in dual sensors then may be best summarized as possibly reducing the false alarm rate (FAR) but not eliminating it. Deminers would still excavate thirty-two times for every mine found. Notably, even when part of a dual sensor, GPR is not the ‘silver bullet’ that can detect and discriminate landmines or other VOEDs. It might best be seen as another tool that can assist in the efforts to find concealed explosive devices in the field that can work alongside tools such as EMI detectors.
GPR for demining is often erroneously deemed to be new technology that was only applied to the problem of finding mines, especially minimum metal mines, from the 1990s onwards.74 This is mistaken. The use of the UHF section of the electromagnetic spectrum in the microwave band to detect mines, specifically minimum metal mines, dates from the Second World War. Both the United Kingdom and the United States devoted considerable research efforts in this direction, with the United States fielding the AN/PRS-1 detector by April 1944.75 The AN/PRS-1 applied radiation in a band of 280 to 350 MHz.76 The challenges of practically detecting mines were well understood with the technical manual stating that “best results are obtained over regular terrain and homogeneous ground. Numerous false indications are caused by ground irregularities, stones, air pockets, roots of trees, pools of water, and nonuniformity of soil.”77 While over 25,000 detectors were produced in 1944,78 quite quickly the AN/PRS-1 had “not proved completely satisfactorily,”79 with “serious limitations"80 and ultimately, despite being “the only type of non-metallic mine detector which offers any possibility of meeting the War Office requirements and a very high priority,”81 its use was discontinued. One of the specific shortcomings identified was not the detection of the increasingly problematic minimum metal mines, but the difficulty the AN/PRS-1 had in detecting S-Mines, bounding fragmentation mines with a large metal content, especially below depths of 3 inches. The AN/PRS-1 did “not find S mines well.”82
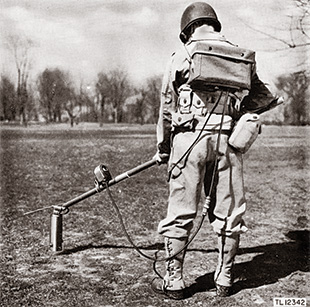
The United Kingdom also looked closely at UHF to detect minimum metal mines. The detector operated at a frequency of 600 Mc/s (600 MHz), and used “CV90 valves in a well-designed microwave circuit.”83 A July 1944 report noted that the prototype detector could, in test conditions, signal for smaller mines such as Schuminen at 2 inches depth, and larger minimum metal mines such as Topfminen at 2 inches, and metallic AV Tellerminen at as much as 12 inches. However, like its US counterpart, it struggled against S-mines, as well as the latest improvised minimum metal mines called picric pots.84
The United States attempted to revisit the use of microwaves for detecting mines in the 1960s. In 1963, the US Army developed the “Microwave Mine Detector” prototype85 but it never saw service during the subsequent Vietnam conflict, despite the heavy toll that mines and booby traps exacted on US and Vietnamese troops. The United Kingdom tried once again, with the Royal Armament Research and Development Establishment (RARDE) conducting trials of pulsed UHF microwaves to detect non-metallic AV mines. A feasibility study into the use of ultrashort RF pulses reiterated that “the problem of detecting non-metallic mines has not yet been solved.”86 Tests showed that saturated soil limited performance and that clay was a harder medium within which to discriminate signatures than sand.87 The research did not produce a functional detector.
The 1982 Falklands War resulted in an estimated 20,000 AP and 5,000 AV mines remaining after the conflict. Ultimately, approximately 11,000 mines would be cleared from 2.3km² of land from 2009 to 2020.88 Initial clearance in the weeks following the war employed 4c mine detectors and prodding. Casualties resulted and the clearance efforts were suspended. The difficulties of clearing minefields in the Falkland Islands led the United Kingdom to re-examine the use of ground penetrating radar using the UHF part of the electromagnetic spectrum. In 1984, the UK government contracted the commercial company Electrical Research Association (ERA) Technology89 to develop detectors that could reliably detect not only the minimum metal mines such as the Spanish P-4-B AP mine but also mines with significant metal content such as the fuze within the Israeli Number 4 AP mine. “The task of clearing the FI minefield is a formidable one for which the Secretary of State has allocated top priority; we have a duty to assess any technique which has any chance of success.”90 Ultimately the ERA ground penetrating radar did not solve the “complex problem of identification of mines”91 and by 1985, research was discontinued. A September 1984 situation report from the Royal Engineers Officer Commanding underlined the problems but also identified the likely line of future progress. “Detection was possible. The problem was sorting out the return signal from rocks, stones, variations in the subsoil, debris etc. The solution to this lies in processing the data from the return signal – so we really seek a mine detector linked to a computer.”92 The implication of this insight is not only the requirement for a database of landmines signatures (and other VOEDs) in various conditions, soil environments, and depths, but also signatures of false positives such as metal fragmentation. Such a database for demining still does not exist today.
In 1996 in the United States, the office of the Assistant Secretary of Defense stated to a House of Representatives Committee that they estimated “that in five years or less, radar using improved signal and data processing techniques should be able to detect anti-personnel mines with a very high level of reliability.”93 The five-year prediction proved optimistic and despite the mine threat in Bosnia and Herzegovina underlining the continuing requirement to reliably detect VOEDs, the United States went to war again in 2001 without a solution to the detection problem. Use of GPR did progress with the deployment of both HSTAMIDS and Minehound dual sensors, as well as Husky vehicle mounted sensors, becoming widely used in military operations in the 2000s. Use for humanitarian demining was more limited to that subsidized by manufacturers or government research agencies. For humanitarian mine action (HMA), often the unit cost was deemed prohibitive relative to any potential increase in operational efficiency, and to date EMI detectors rather than dual sensors remain the standard detection technology within the demining sector.
Mine Detection Dogs
Over the past eight decades significant hope has been invested in the potential of mine detection dogs (MDD) to be an answer to the detection problem and some still advocate that MDD can be a completely effective and reliable detection tool. There is ample evidence over many years that dogs can detect concealed explosives, most probably by some form of scent indication,95 although this has never been categorically proven. Research in 1947, where four trained MDDs had their olfactory nerves surgically removed and yet still indicated on concealed mines, points to the possibility of other senses being employed.96,97 However, the reliability of dogs has often been questioned and it is accepted that dogs are not good at indicating the individual position of mines within a minefield, possibly due to a confused scent picture. The most recent international standard accepts that MDD “are best at working in areas where there are low concentrations of explosive ordnance.”98
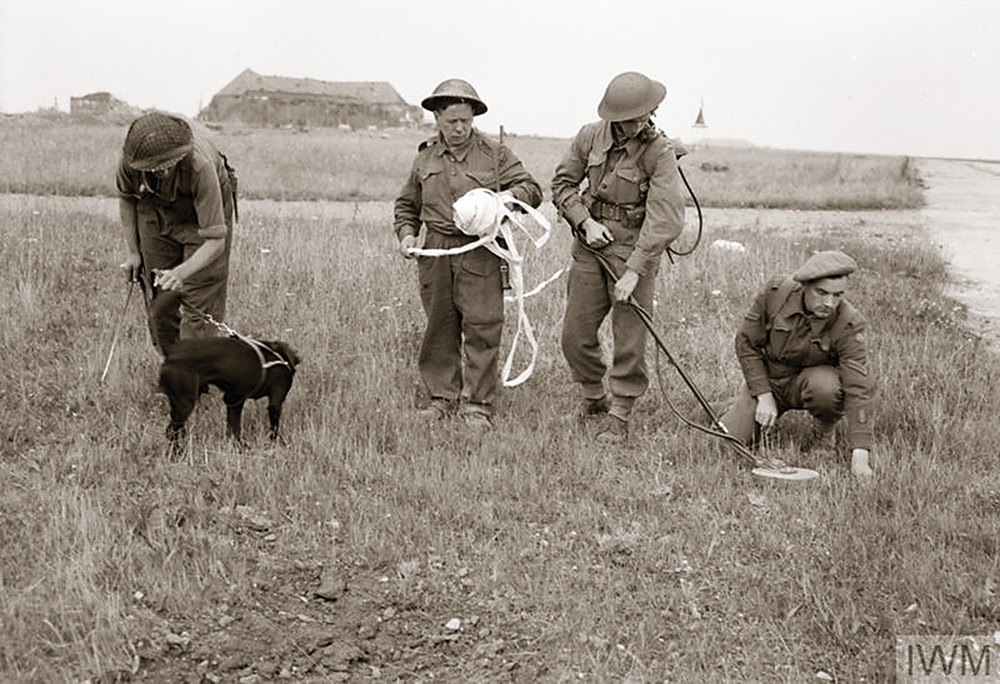
The use of MDD was first attempted during the Second World War. The British started to encounter more mines that by the standards of the day were deemed “minimum metal.” These included the wooden cased Schützenmine 42 and the Holznminen 42. The ZZ-42 cocked striker igniters used with both included a steel striker, spring and holding device, significant metal content by later standards but difficult to practically detect at the time.99 In early 1943, trials began in earnest at the Obstacle Assault Centre (OAC) in Aldershot, United Kingdom. During one comparative test, dogs found nine out of ten mines on a 1 kilometer stretch of road where a Sapper operating a PMD model located only four. On this basis, four Royal Engineer Dogs Platoons were formed in April 1944 for deployment in Northwest Europe.100 The results were mixed. An initial task to search Carpiquet Airfield near Caen in July and August 1944 saw the dogs struggle with an area where intense combat had very recently occurred. The heat and dust were deemed particularly difficult. Mines were missed and the dog platoon commander lost a foot.101 As the platoons moved into the Netherlands, their use became more focused on defining general areas where mines were rather than specific locations of individual mines. Dogs were also deemed useful for defining where mines were not, or at least giving a degree of reassurance in this respect. Critical infrastructure such as roads and rail lines could be interdicted by a handful of mines along a distance of many kilometers. In the absence of manpower to slowly clear these long stretches, dogs provided a means of rapid search. The US military also deployed dogs, referred to as M-Dogs,102 to the Fifth Army in Italy. Initial hopes as to effectiveness were extravagant, with the War Department Technical Bulletin claiming that an M-Dog “will detect all buried objects within a given area, working in daylight or darkness, over any sort of terrain, in any weather.”103 Over the next few months, M-Dog use was deemed unsuccessful and the unit was disbanded within a year.104,105
Research continued after the war with anatomist Sir Solly Zuckerman in the United Kingdom, who eventually concluded that dogs were not suitable for practicable landmine detection.106 During the Vietnam War, the increasing casualty rate sustained due to mines and booby traps was a catalyst for the US military to try again with dogs as a means of detection from 1969 onwards. A 1971 assessment, “Mine Dog Successes and Failures” outlined the mixed record of their deployment. For example, “on 14 April, King 3K92, cleared a road for A Company, 39th Engineers. King alerted on an undisturbed portion of the road. A mine detector team failed to detect anything in the vicinity of the alert. However, the area was marked and blown by EOD. A subsequent secondary explosive resulted and was estimated to have been caused by a 30–40 lb non-metallic mine.” However, the same report pointed out serious failures. “On 14 April, the 21st APC of a mechanized convoy from B/1-61, detonated a 20 Ib metallic anti-tank mine after the road had been cleared by a mine dog, Abby, 7K39, and an Engineer mine team.”107
Despite a mixed record, and unlike the Second World War, the US military continued with dogs. The Field Manual 7-41 Mine and Tunnel Dog Training and Employment was published in March 1973108 and there was significant research in the 1970s to better understand how dogs could detect explosives. One 1974 study, echoing that of the British in 1947, suggested that dogs responded to a range of cues including ancillary human scent and disturbed earth.109 Later trials at Fort Belvoir emphasized the importance of the dog handler relationship if the use of MDDs was to be effective. The combination was believed to include the handler’s cognitive ability to recognize various mine and booby trap indications, along with their visual sense, and the dog’s ability.110 Such thinking endured, with a 2004 US military study stating that an MDD was a means to “produce a highly sophisticated and versatile extension of a soldier’s own senses.”111 Research in 1977 compared MDDs with searchers equipped with the AN/PSS-11 EMI detector. The results were mixed. It was found that experienced soldiers with AN/PSS-11 outperformed MDDs when searching for M-15, M-16, and M-18 mines with high metallic content but that MDDs were superior when searching for minimum metal M-14 and M-19 mines. While the AN/PSS-11 detector notably outperformed MDDs during winter testing when there was snow on the ground, in general it also had “a false alarm rate much greater than that for the dogs.”112
Today, dogs are still used by both military and humanitarian demining and EOD teams. In 2011, Lt. General Michael Oates of JIEDDO still believed that dogs combined with handlers were amongst the best means of detection available to the US military113 High assurance search dogs became an important tool in the counter-IED effort. Some demining organizations remained skeptical, with one claiming that in Herat, Afghanistan, in 2015, a number of accidents resulted from mines missed by dogs.114 “MDD have a poor record in Afghanistan for clearing AVM. They were used in Jebrail in Herat Province where minimum metal mines were missed. Although numerous reasons have been identified for the mines being missed, the environmental conditions in Afghanistan are challenging for MDD and tests have shown that their performance can be inconsistent.”115 For this reason, while MDDs are still deemed to have an important role, as in the 1940s, they are not the solution to the detection problem, but one more tool amongst others. As a US Army Engineer report concluded after a visit to the British War Dog Training Centre in March 1945, “no dog can guarantee to work perfectly at all times.”116 Six decades later in 2004 another US military report concluded that, “MDDs are not a stand-alone system for conducting mine clearance operations.”117
Prodding and Full Excavation
While rudimentary, the detection problem still leads to the most basic methods being employed, at least for mines: prodding and full excavation. Prodding was recently one of the first detection techniques used and was widely employed during post-war clearance, often resulting in significant casualties when clearing sensitive pressure devices such as AP Schumines.118 One 1946 US military review of metal detector technology stated that the “effectiveness” of prodding “was the major reason for discarding a number of mine detector developments” during the war.119 In an Infantry conference in Fort Benning in the same year it was stated that “the most satisfactory means of tactical mine removal presently available for infantry troops is probing, hand removal of mines.”120 Sixteen years later in 1962, a UK Royal Engineers pamphlet stated that “prodding is a slow and laborious process, but it is the only method capable of detecting all types of mine buried at normal depths; it has therefore become the standard method of detecting mines.”121
Prodding remained an accepted military detection technique for decades. In Bosnia and Herzegovina in 1994, the British Army taught prodding for shallow mines and the employment of detectors only for mines suspected to be deeper.122 The technique can still be taught as a last resort for those who might inadvertently find themselves in a mined area. More recently prodding has been questioned as an advisable method due to the risk of inadvertently initiating pressure operated AP mines and the limited depth of effective search it provides.123 Stones and roots will provide frequent false positives. Prodding was used in the Falkland Islands for searching some AV only minefields. However, as a means of area clearance, it has largely been overtaken by full excavation, especially if the use of EMI detectors is not deemed practical due to high levels of metal contamination or laterite soil.124 It can also be used during individual target investigation. If the explosive hazard is relatively small, such as associated with AP mines, and the ground is sandy, rakes may be used to conduct full excavation. While there is a risk of initiating items during the process of raking, the distance between the deminer and any detonation, combined with personal protective equipment that conforms to standards, makes the consequences of such an AP blast mine detonation less severe.
Visual Detection
It might be argued that the best detector over the past eight decades has been the human eyeball combined with cognitive ability to interpret what is being seen. Consistently, both anecdotal evidence and such limited data that has been collected tends to point to the enduring, albeit limited effectiveness of human vision combined with knowledge of VOED indicators. Various electronic detectors such as EMI, GPR, or dual sensors have played a role, as have MDDs, but all rely on a combination of human vision and cognition. “Even with all the investments in detection technology, an observant human eye detects the vast majority of IEDs” (of those detected).125
The relative effectiveness of visual detection has been appreciated since the 1940s. In February 1945, No.2 Dogs Platoon Royal Engineers were tasked to search a suspected minefield in the Netherlands. Over three days, the platoon found 112 picric pots, (a German improvised mine). However, another 433 were visually identified by handlers or other sappers. While it was also true that the dogs had not been trained on picric scents, in the task report the Platoon Commander noted that “only signs, visible mines and knowledge of pattern saved handlers from treading on mines.”126 The officer commanding the attached Royal Engineers Field Company believed that the ability to recognize the pattern from visual cues made his men 50 percent more efficient than the dogs at finding mines.127 This was consistent with experience from earlier in the campaign.128 A 1963 US military manual stated that “visual detection is one of the best and most effective methods of locating mines embedded in most types of soils. Indications of buried mines are disturbed soil, piles of stones, debris from mine packaging, and often enemy minefield markers.”129
The primacy of visual detection was emphasized repeatedly during the Vietnam War. A 1969 US Marine Corps analysis stated that “although a great many detection means, ranging from intricate electronic devices to specially trained dogs, have been developed, experience has shown that an alert marine, aware of what to look for and where to look, is the most effective detection device.”130 A 1969 9th Infantry Division study of both mines and booby traps stated bluntly that “almost all of the booby traps are detected visually. Very few, less than one percent, have been detected as a result of informants or scout dogs. Therefore, men should be constantly on the alert, eyes focused and searching ahead of the line of march. In this respect training is of vital importance to new men in-country prior to gaining the experience that comes only with being in the rice paddies.”131 A 1974 post-war study estimated that 60 percent of mines and booby traps detected in Vietnam were “by visual or related means.”132 Another 1974 analysis claimed that “reliance on perceptual detection of mines by man is empirical fact. Reports from South Vietnam and previous conflicts indicate that 70 to 80 percent of all mine detections are attributable to unaided personnel.”133 The British, analyzing the “urgent” problem of nuisance mining in Aden in the mid-1960s recognized that they relied on visual sweeps to initially detect mines on routes.134
Between 1973 and 1974, recognizing how during the Vietnam War mine and booby traps caused high casualties and limitations on how ground troops maneuvered,135 the US Army engaged the Human Resources Research Organization to research how troops found such devices using their own senses. Seventy-eight individuals were tested and it was believed that tests showed “detection expertise probably is an acquired skill rather than an aptitude-orientated skill.”136,137 Subjects indicated that while they would prefer other detection means such as metal detectors and MDDs, they would see these as “supplements rather than substitutes for visual detection.”138 Tests in 1974 indicated that color and shape, along with quality of camouflage, were important factors in determining the visual detection of devices.139 The US military conducted further tests in 2008 to try to understand what visual cues were perceived by subjects known to have a level of expertise concerning a mine threat. Of note was how “expert perceivers” noticed changes in soil structure.140
In October 2009, General Thomas Metz, the head of JIEDDO, testified to the US House of Representatives Committee on Armed Services that “both inherent traits and experience-related characteristics proved essential to IED detection performance. Our results suggest that IED detection is largely a cognitive task, relying on visual, attentional, and memory processes. One significant finding that emerged indicates certain hobbies are relevant to successful IED detection. Specifically, participation in hunting, puzzles, art, and music predicted superior performance, as did time in service, deployment experience, and age.”141 In 2011, the US military conducted research into “perceptual learning to improve the visual detection of IEDs.”142 How much such skills are related to natural aptitude and how much they can be acquired remains unclear. In any case, in November 2012, a US Army research laboratory article stated that “despite its investment in millions of dollars’ worth of sophisticated technology, U.S. Army’s researchers say visual detection is the primary means of discovering Improvised Explosive Devices.”143 In 2007, one journalist claimed that “soldiers who once spotted few roadside bombs in Iraq now detect more than half before detonation. The ‘Mark 1 Human Eyeball,’ as troops sardonically call it, is more adept at finding IEDs than any machine.”144 Much of the training of those in threat environments concentrated on increasing their perception to improve visual detection of IEDs,145 often through courses such as Ground Sign Awareness.146 Even in 2020, one HMA organization stated that “visual detection is one of the primary techniques to identify IEDs in any environment.”147
It is possible that a sensor technology will prove to be a viable solution to the detection problem. Research to this end continues. However, if it is accepted that vision combined with cognition remains a significant albeit limited element of detecting some VOEDs, practically developing that cognition should be a priority. What this entails is better informed personnel on the ground who can more often successfully interpret what they see. This means equipping those individuals with contextual knowledge based on evidence rather than intuition or anecdote. In order to provide that contextual knowledge as accurately as possible, it is necessary to actively gather as much relevant operational data as practicable. The significance of a given visual cue can be better calculated if the number of times it has previously indicated the presence of a VOED is recorded. Essentially, visual detection combined with knowledge entails an individual making a form of qualitative risk assessment on the ground. That risk assessment should not only be informed by personal experience, but also by quantitative data. For example, mines being frequently placed on verges or near culverts might be believed to be true due to personal experience or anecdote, but it is better if based on operational location data that has been carefully collected over time. As with other means of detection, routinely recording and analyzing how previous mines were found can better inform future efforts.
Unexploded Ordnance Detection, Discrimination, and Classification
Related to the problem of detecting VOEDs is the more general problem of detecting unexploded ordnance (UXO).148 The base closures in the United States at the end of the Cold War led to greater prominence of the issue of removing UXO.149 Detecting UXO with high metal content was possible depending on the size and depth of target. However, discriminating UXO from metal “clutter” and other issues such as the “geological noise originating from magnetic soils”150 meant that the number of false positives made clearance expensive and slow. Increasing the sensitivity of EMI sensors to find smaller items at greater depth also resulted in a greater FAR.151 Magnetometers were also widely employed and remain useful for large items that are deeply buried. However, the recorded magnetic data has limitations on measuring the size and shape of a target and therefore magnetometers are not suitable for most discrimination purposes.152
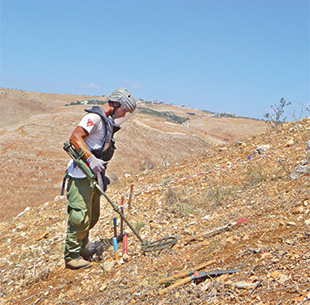
The inability to discriminate UXO from clutter and the clearance costs this entailed was referred to as “one of the Department of Defense’s (DOD) most pressing environmental problems.”153,154 Significant research and development efforts have enabled some degree of Advance Geophysical Classification (AGC) of UXO, dependent on multi-axis sensors rather than the single-axis sensors associated with demining.155 The former required a number of EMI sensors arranged in an array. These measured the polarizability of a target; that is the electric dipole moment in proportion to the electric field applied. This could be recorded and act as a reference for future comparison.156 To a degree this allowed discrimination between scrap metal and UXO although the results should not be overstated. The real result was a reduction in the number of false positive signals to be investigated157,158 in a way analogous to the use of dual sensors searching for AP mines in Cambodia.159 AGC is not applicable for mines with little or no metal content. The potential wider application to mined areas is also limited due to the need to remove excessive vegetation prior to the sensors covering the ground,160 even if the sensors are mounted on an unmanned aerial vehicle (UAV).
While AGC has assisted in the removal of UXO from contaminated sites in the United States, it should not be seen as a silver bullet. One impediment is the aging of EO causing change in the polarization of items to such an extent that they cannot be classified. Partial UXO fragments that don’t conform to a pre-recorded signature but nevertheless still represent an explosive hazard are also a problem. Any artificial intelligence (AI) processing of signatures depends on comparison with a predefined library of polarizability signatures.161 Presently such databases are in their infancy. The creation of such databases for EO initially requires a human to excavate and confirm visually what each signal represents. For UXO in the United States, the lack of signature data to inform operators of what they are looking for on a given range, especially for munitions that did not graduate into production, remains a significant impediment.162 The need to develop a dataset of target signatures for comparison is clear. Any technological advances to enable discrimination and classification of targets, whether it is UXO or VOEDs such as AP mines, depends on developing such a dataset. This requires data entry by clearance operators to confirm not only the identity but also other relevant information about each item of EO. Progress on the longstanding detection problem, both in technological and operational risk management terms, may be said to depend on the collection of operational data.
Other Attempts at Technological Solutions
Aside from EMI detectors, and use of GPR and MDDs, many research attempts at solving the detection problem have been attempted over the past eight decades. In the mid-1960s, the British Army conducted airborne trials in Salisbury Plain, Borneo, and Aden. The trials, known as Operation FRICTION, used infrared systems called Linescan and Side Looking Airborne Radar (SLAR). The trials showed that identifying individual mines was impracticable and identifying patterns was only possible in certain circumstances if a survey of the area prior to the emplacement of a minefield was available for comparison.163 Minefields that were overgrown by vegetation, as is often the case, could not be detected by these means.164 (Vegetation is a stubborn impediment for all sensors mounted on piloted aircraft or UAVs.) The trials were deemed urgent since “in South Arabia there is a persistent and serious threat from dissident mining” causing significant military and civilian casualties. The existing methods used were “handheld detectors or visual sweeps. These methods are slow, inaccurate and unsuitable for covering long distances.”165 Today, the military use a technique called Coherent Change Detection (CCD) that compares time lapsed high resolution satellite or UAV images to try to identify relevant change.166 While a useful tool, the technique applies more to a changing tactical environment rather than long-term contamination. Use of thermal imaging as a tool to potentially indicate explosive devices concealed in the environment continues to the present, whether as a tool to try to identify IEDs on military operations,167 or as a means of identifying mines in desert environments.168,169 The use of thermal imaging, while having a degree of utility in certain environments, has yet to show potential as a reliable means of detection in all environments.
Use of acoustic waves was subject to research in the 1940s170 and in 1973, the need for a means of detection that “does not depend significantly on the materials from which the mines are constructed” was identified.171 Seismo-acoustic methods work by detecting vibrations in a device that are generated and received by a pair of transducers. Tests showed that a 3 KHz signal reflected from simulated mines down to 12 inches.172 More modern efforts use a frequency range of 50 Hz to 1KHz.173 However, the technology required a vehicle mounting, limiting its practical use. It has also been deemed more suited to AV mines rather than smaller AP mines.174 For a time, Nuclear Quadrupole Resonance techniques were deemed as having potential by means of directly identifying the nitrogen molecules used in common explosives such as RDX. Identifying TNT proved more difficult, as did metal encased mines.175 Development of this chemical analysis technique is still being attempted today in Colombia, but even in test conditions the claimed range a substance can be detected is only 3cm.176 Recent efforts on the related magnetic resonance technology have not progressed to independently verified field trials.177
In the absence of reliable means to detect and discriminate devices, sometimes detection by detonation has been employed. Rollers have been used on routes since the Second World War,178 with limited success due to uneven ground and the potential orientation of the means of initiation of the VOED.179,180 Flail attachments have been deployed as a means of detection by detonation since 1942, but also depend on the orientation of the mine and the condition of its fuzing system. Flails can also struggle in uneven or steeper ground and can throw items rather than initiate them.181 Soldiers have also resorted to throwing drag weights on pull lines to try to detect trip wires in Vietnam.182 In both Iraq and Afghanistan, it was realized that it was easier to detect the human being emplacing a VOED than the device itself, and this became a recognized method of IED detection.183
Sometimes means other than detection have been used to try to reduce the risk from potential contamination. In Vietnam, the US military routinely bombarded landing zones prior to infiltration to try to reduce the risks from mines and booby traps.184 Fuel Air Explosive was used to clear not only any vegetation, but also any pressure initiated VOEDs.185 Minefield breaching operations still use explosive line charges projected into an area to at least partially clear a path.186,187 These might be supplemented by a mine plough that shifts any potentially contaminated earth away from a breaching lane. Such charges were even used during counter insurgency operations in Sangin, Afghanistan, in 2010.188 During the post-conflict clearance in the Falkland Islands, such was the difficulty of the detection problem that consideration was given in late 1984 to the use of Fuel Air Explosive to clear areas of land and therefore bypass the difficulties of detecting minimum metal mines.189 Trials were conducted in Canada but the technique was never tried on the Falkland Islands.
The Approach to the Problem
In December 1978, the First Meeting of the Expert Working Group (EWG) on Minefield Detection was held in the United States. In attendance were the US Army’s Mobility Equipment Research and Development Command (MERADCOM), Environmental Research institute of Michigan (ERIM), and the defense company Braddock, Dunn, and McDonald (BDM). The participants recognized that “the function of the Expert Working Group is to provide broad technical and operational guidance on the mine detection research effort. The EWG should determine that the problem to be solved is realistically understood and assessed, that the effort is accurately directed toward this problem, and that no significant aspect of the problem is being overlooked.”190 The working group did not last, and the problem was not solved. Unfortunately, the approach outlined did not describe the overall approach to the problem over the last eight decades. Often it is not well defined, with much research wasted for lack of a true appreciation of realities in the field.191,192 For example, vehicle-based sensors limit the practical application of any system since even tracked vehicles have limited mobility over much of the terrain where landmines are found, and yet such sensors are still promoted as practical solutions.193
In 1996, the US General Accounting Office (GAO) identified significant failings with the US Army procurement of its mine detectors. After a process that considered twelve candidate pulse induction and continuous wave EMI devices, a model from the Austrian manufacturer Scheibel GmbH was selected in December 1991 and designated AN/PSS-12. The GAO subsequently remarked that Scheibel did not perform well during testing against low metallic targets but was selected on price considerations. The testing itself was deemed inadequate. Since no candidate detector could detect low metal targets, steel content was added to targets in order to pass the test.194 The remarkable aspects of this are not necessarily the questionable procurement process. What was notable was that the continuing “detection problem”195 was essentially ignored, partly, it might be assumed, because there was no real answer. The detector was fielded on the basis that it could detect a range of mines, including those with low metal content, but practically it could not. Ultimately the AN/PSS-12 would be the subject of significant redesigns to try to improve its performance. These included a new algorithm and hardware to try to increase discrimination performance to discern clutter from positive signals.196 Even today some EMI detectors are marketed as being “capable of detecting all currently deployed minimum metal AP and AT mines.”197,198
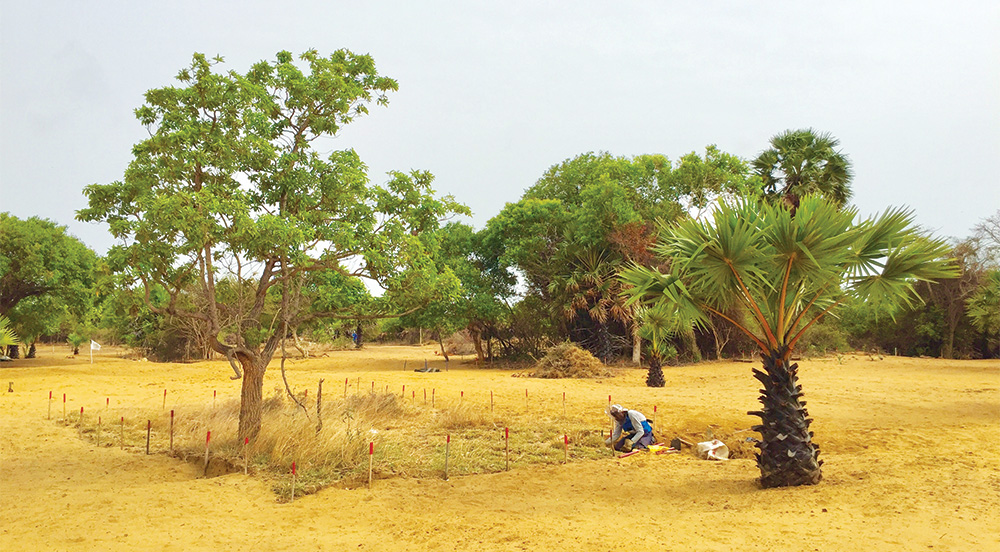
While there was an understandable focus on minimum metal mines, the truth was and remains that in conditions of metal contamination, which are inevitable in areas where combat has taken place, reliably discriminating all items of EO, regardless of metal content, is not currently possible. This fundamental aspect of the problem has been known about for years but has not necessarily been appreciated when it came to detection research efforts. In Vietnam, booby traps incorporating significant metal content for explosive fragmentation effects were the biggest problem for US ground troops. A leaflet distributed to 9th Division Troops in May 1969 stated that “in April 1969, 41% of the 9th Division soldiers killed in action, and 63% of those wounded were casualties from booby traps. Booby traps are the single most important casualty producer in the 9th Division area.”199 Of the booby traps themselves, an earlier assessment noted that 71.3 percent used a grenade, and another 11.8 percent used an artillery projectile or mortar round, usually initiated by a tripwire. All these are items of EO with significant metal content.200 This is not to say that minimum metal mines, referred to as plastic mines, were not a challenge. The same 9th Infantry Division noted in 1968 that “new enemy techniques encountered were the increased use of hard to detect plastic mines in place of metal mines and the construction of numerous small roadblocks in clusters. Within each cluster, several are booby trapped. Since any of them might be rigged, each one has to be painstakingly cleared which increases the time expended opening the road.”201 Regardless of metal content, detection of mine and booby traps remained a problem. For the calendar month of April 1969 in the 9th Division TAOI,202 only 1.6 percent of mines and booby traps were detected by metal detectors.203 The figure for May 1969 was even lower at 0.3 percent.204 For a three-month period (March to June) in 1968, metal detectors found 3.9 percent of identified booby traps, whereas 40.9 percent were identified visually and 51.3 percent were identified through detonation, and hence too late.205
The detection problem has been partially recognized, albeit perhaps obliquely, by international treaty. The 1996 Amended Protocol II of the Convention on the Use of Certain Conventional Weapons required that AP mines must be detectable using commonly available mine detection equipment, which effectively banned minimum metal mines.206 The convention was only enforceable for states that were high contracting parties to it. Today this totals 106 countries.207 While effectively trying to ban minimum metal mines, the overall problem of detecting all AP mines in operational conditions, regardless of metal content, would only be addressed by an outright ban of victim operated AP mines brought about by the 1997 Anti-Personnel Mine Ban Convention.208 One hundred and sixty-four states are party to the Convention.209
In November 1996, Major General Clair Gill, commanding the Engineer Center at Fort Leonard Wood, stated to a symposium on technology and the mine problem that “in the very near future, anyone that would bury a container filled with explosives should have the absolute certainty that it will be found and neutralized with little effort and at no operational expense by U.S. ground forces. With detection and neutralization so easy and certain, I’m confident that the threat of the landmine will wither away.”210 Despite this optimistic prediction, the United States would begin the conflicts in Afghanistan and Iraq with “mine detection capabilities that are limited and largely unchanged since the Second World War.”211 In April 2001 it was again noted that not only did the detection problem persist but that the approach to the problem remained inadequate. A GAO report asserted that the Department of Defense lacked “an effective strategy for identifying and evaluating the most promising technologies.”212 Notably the report recommended that evaluation of “all applicable land mine detection technologies against a complete set of mission-based criteria, such as target signatures, operational requirements and expected environmental conditions.”213 In short, research should be applicable to the problem as it was experienced in the field. Despite this observation, a US military doctrine of assured mobility evolved over the 2000s. “Assured mobility is the framework of processes, actions, and capabilities that enable the joint force to deploy and manoeuvre where and when desired, without interruption or delay, to accomplish the mission.”214 When viewed through the lens of coalition experience in Afghanistan and Iraq, it is not clear whether such a doctrine was practically achievable, or based on a sufficient understanding of the problem, given the ongoing difficulty in detecting and discriminating victim operated IEDs.
While research and development (R&D) efforts to find a technological solution will and of course should continue, the difficulty of the problem should always be clearly understood. To accurately identify different substances in the ground is more of a scientific challenge than many might realize. The real requirement is for a sensor that can not only reliably detect VOEDs and discriminate them from false positives, but also work in a range of environmental conditions, including levels of vegetation and soil types. For VOEDs, such sensors should be reliable down to typical search depths (perhaps 20–30cm). Those seeking to engage with the problem should understand that they are attempting to find a solution that has eluded considerable efforts over more than eight decades, including extremely well-funded efforts conducted as a very high priority during conflict. R&D projects should resist the temptation to overclaim perceived future success, even though the need to attract funding may explain such marketing. Any R&D project seeking funding should clearly outline how their technology will engage with the problem as it is experienced by clearance organizations in the field, and also why this particular technology has the potential to succeed where so many attempts in the past have come up short.
Possible Areas of Development
Many attempted detection research efforts have sought to remove the human from hazardous areas during the process of searching for mines, booby traps, and victim operated IEDs. Historically, armored vehicles were employed as platforms for sensors, and these vehicles progressively became remotely operated. More recently, UAVs have been widely employed, with increasing attempts to mount a range of sensors and analyze a fusion of the results. Unfortunately, these efforts have two fundamental impediments. Firstly, mined areas are often, at least in temperate or tropical environments, covered with vegetation that will be a key impediment for EMI, GPR, infrared and even LIDAR sensors. If the topography permits, such vegetation can be removed by a machine, although often it is removed by a deminer. The second impediment is that regardless of the sensor employed, and the means by which it is employed (UAV, armored vehicle, deminer), the identity of a sub-surface signal can only be ascertained by a deminer excavating it. A sensor might indicate the potential presence of an AP mine but only a deminer can excavate it to provide final confirmation. No sensor can categorically identify which signals may be ignored and which merit excavation; therefore, deminers will continue to be restricted to slow, deliberate searching of ground, even if the false positives investigated may to some extent be reduced by dual EMI and GPR detectors.
What could overcome this problem is the development of a database of signatures of the range of VOEDs for a range of sensors. However, in order to develop such a database, clearance organizations will have to systematically record not only the signature of every hazard found for a given sensor, the settings of that sensor, the details of the explosive hazard, and the environment it was found in, but also the equivalent detail for false positive signatures. The road to AI rapidly discerning whether a given signal is a real device or not possibly runs through the laborious collection of signatures against which any new signal can be compared.
Conclusion
In terms of detecting and discriminating VOEDs, the last eight decades may be seen as largely a history of failure, or at least one of insufficient success.215,216 Many technological solutions have been proposed, but all have struggled to perform in operational conditions where the challenge is not only to detect an object by its metal content, differing density to the surrounding soil, chemical composition, or heat signature, but also to discriminate it from other false positives that may be detectable, such as scrap metal, rocks, etc. The detection problem is not limited to mines with low metal content, although this is perhaps its most acute aspect. Even EO such as a mortar round with easily detectable metal content is often difficult to practically find when concealed beneath the surface, not because it is hard to detect, but because it is hard to discriminate from much else. In military scenarios, detection has remained a form of mitigation since it is only ever partially effective. In humanitarian scenarios, the lack of time constraints means that while detectors have a significant, arguably crucial role to play, their effectiveness remains limited to such an extent that clearance of land is slow and laborious, and often the clearance solution remains the least technological: full excavation of ground.
In 1971, the Assistant Division Commander of the 1st Marine Division concluded after their two-year deployment in Vietnam that “the 1st Marine Division’s strenuous efforts – including troop indoctrination, land mine warfare school, contact teams and mine and booby-trap dogs – did not solve the problem. The best we can conclude is that these efforts greatly reduced what might have been the casualty figures if they had not been vigorously pursued.”217 Today, as in 1971, mine detection technology, combined with other procedural forms of mitigation such as training, can only achieve so much. Technological R&D might assist, but so far has produced no silver bullet. The detection rate for concealed IEDs in Afghanistan remained at approximately 50 percent, despite the huge investment by JIEDDO since 2006.218 Until a technological solution can be found, the question remains how to make such mitigation as effective as possible. Finding and destroying concealed VOEDs is a risk activity, and conducting such activity with the limited detection capabilities currently available emphasizes the risk management characteristic further. If the ISO definition of risk as the effect of uncertainty on objectives is accepted, concealed VOEDs represent an acute uncertainty that has affected both military and humanitarian objectives.219 Therefore, in the absence of a comprehensive technological detection solution, more effort may be placed on enhanced risk management approaches to mitigate the risk as best as practicable. Such approaches should inevitably be based on the collection of as much accurate operational data concerning VOEDs as practicable, including not only their identity and characteristics but also how and where they were found and destroyed. Such an approach does not represent any sort of a complete solution, but it is an available means of reducing uncertainty, and better managing risk.
See endnotes below.
Roly Evans has worked in the fields of survey, clearance, EOD, and physical security and stockpile management in Africa, Europe, the Middle East, South Asia, and Southeast Asia and is conducting research on mine action operations. He holds an MSc in Explosive Ordnance Engineering.
Tracey Temple, PhD, began her career in the RAF and then went on to study as a mature student where she graduated from Queen's University, Belfast (2002) with an Honour’s degree in Geography (2002) and later a Master of Science in Rural Development and Project Management (2004). In 2017, Temple completed her PhD at Cranfield University in the fate and transport of explosives in the environment.
Lt Col (Retd) Liz Nelson is a lecturer in Explosives Technology and Safety at Cranfield Defence and Security, Defence Academy of the United Kingdom. She is also the CDS Explosives Safety Advisor. Lt Col Nelson has over thirty years’ experience related to ordnance, munitions, and explosives safety and technology from her time as an Ammunition Technical Officer in the British Army. She has a Master of Science from Lincoln University and joined Cranfield University in September 2022.